XCITE Lab research
Overview of projects
We are actively working on a number of projects briefly listed in the table below. Most of the projects are investigated by computer simulations as well as experimentally. We are lucky enough to have two fancy lab spaces. The first lab features a table-top x-ray imaging system for computed tomography investigations. The second lab features a dual-robot system and it is used for investigations of x-ray radiation therapy. Apart from research conducted directly at UVic, we have experimental collaborations with CERN in Geneva, TRIUMF in Vancouver, and ALLS in Montreal in the near future hopefully! In terms of computer work, we are collaborating with RaySearch in Stockholm, Redlen Technologies in Victoria and Mayo Clinic in Rochester. We also work with Medscint and Laval University on plastic scintillator dosimetry.
Project | Lab members | Spatially-fractionated radiotherapy/FLASH | Nolan Esplen, Alex Hart, Jade Fischer, Nathan Clements, Maddie Perry, Jon Eby |
---|---|
Kilovoltage Optimized AcceLerator for Adaptive (KOALA) therapy | Dylan Breitkreutz, Jericho O'Connell, Olivia Masella, Nathan Clements, Sandhya Rottoo, Jacob Atkinson |
X-ray imaging with photon-counting detectors | Pierre-Antoine Rodesch, Devon Richtsmeier, Jericho O'Connell, Kevin Murphy, Joanna Nguyen, Alison Deng, James Day |
Small animal radiotherapy | Chris Johnstone, Nolan Esplen |
Spatially-fractionated radiotherapy (SFRT) and FLASH
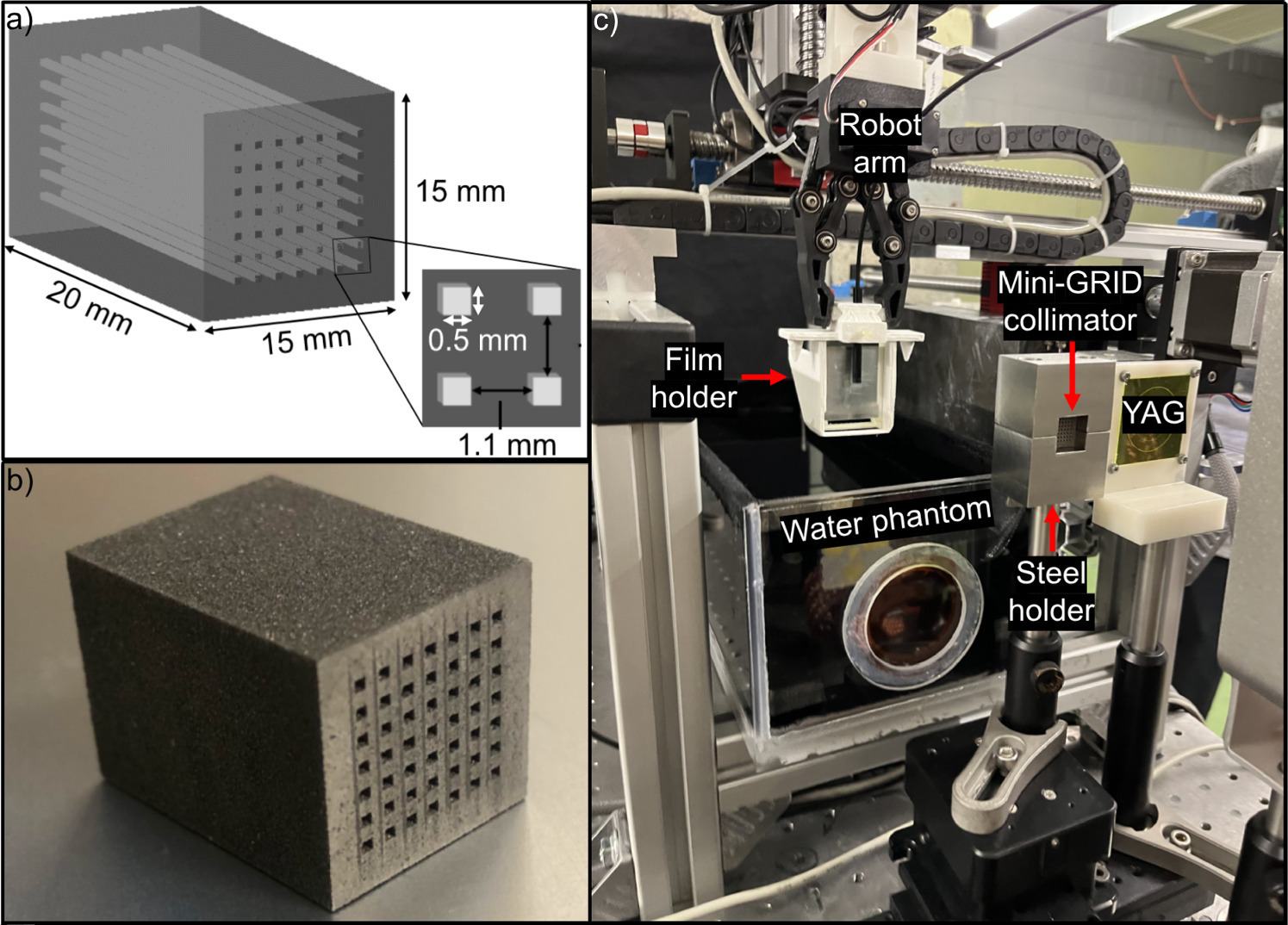
FLASH and spatially-fractionated radiotherapy (SFRT) have shown great promise in the decrease of treatment side-effects while maintaining the potential to kill tumor cells. SFRT has been implemented in the form of microbeams, minibeams of grid therapy, mainly on synchrotron sources with limited access. We are investigating the feasibility to deliver SFRT on the SARRP system. We have built a custom collimator that can be inserted into the SARRP 10x10 mm nozzle and results in peak-to-valley dose ratios (PVDRs) of ~13 and isocenter dose rates of ~ 1.2 Gy/min. We have also tested two other 3D-printed tungsten miniGRID and microGRID collimators for SFRT dose delivery on the SARRP. The microGRID collimator with 400 μm slit size and 287.5 μm center-to-center distance resulted in high PVDR values of 58. We have also used the CERN CLEAR beamline for the delivery of SFRT with very high-energy electron (VHEE) beams. We have done this in three different ways: by using a tungsten grid collimator and by pencil beam scanning delivered either by stationary pencil beam and moving the object in 2D or by directly scanning the pencil beam. The critical parameter PVDR was the highest for pencil beam scanning and decreased for object scanning and even further for irradiation performed with the miniGRID collimator. We are working with RayStation to assess VHEE SFRT treatment plans.
We demonstrated that conventional x-ray tubes are capable of FLASH dose delivery and published on this as a Medical Physics Letter. We have built a custom beam shutter to alow for irradiations as short as 1 ms and have used this system to evaluate a number of organic, inorganic and hybrid scintillator dosimeters for ultrahigh dose-rate dosimetry. We have also optimized the shutter system for uniform dose delivery across a 6-mm diameter sample. We investigated the survival of fruit flies after ultrahigh dose-rate (UHDR) delivery and demonstrated that there is a dose window in which increased emergence and extended fly survival exist after UHDR irradiation compared to conventional irradiation. The next step will involve irradiations of 3D printed normal and cancerous skin cells, a project that we are working on in collaboration with Dr. Willerth's lab at UVic.
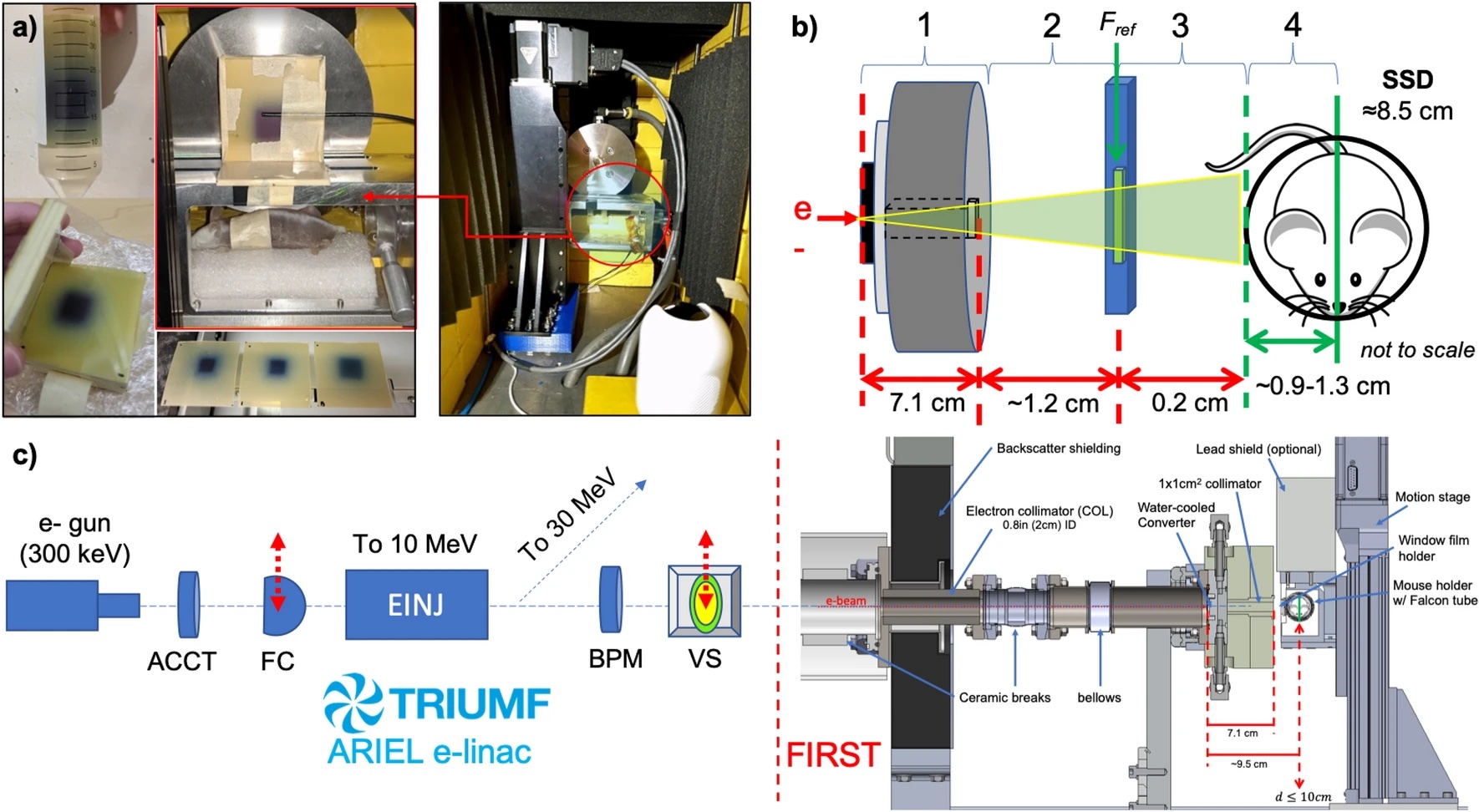
In addition, we built a FLASH irradiation station at the ARIEL beamline at TRIUMF, a high-energy physics laboratory in Vancouver. By means of heat transport simulations, we have designed an electron-to-photon converter for the delivery of an ultrahigh dose-rate 10 MV photon beam on the existing medium energy beam dump. Our beam modeling suggests that the achieved dose rates at the irradiation site should be up to 200 Gy/s and above the FLASH effect dose rate threshold of 40 Gy/s to depths of 10 cm. Additionally, thanks to Monte Carlo simulations with the TOPAS code, we have designed a GRID collimator for the delivery of SFRT with PVDR of ~5. The aim of the NFRF-E funded project is to investigate the FLASH effect for open and spatially-fractionated beams on healthy lungs of mice with the 10 MV beam. After 2.5 years of designing a target to convert high-flux 10 MeV electrons to 10 MV photons on the TRIUMF ARIEL beamline, we started our FLASH irradiation station installation in October 2021 and completed it in March 2022. Later in 2022, we performed mouse irradiation experiments to investigate the FLASH effect with ultrahigh dose rate high-energy photons. We did not observe the FLASH effect, but learned all the aspects we can improve in our experiments.
Related publications- Fischer J, Whitmore L, Sheehy S, DesRosiers C, Bazalova-Carter M, Very high-energy electrons as radiotherapy opportunity, EPJ Plus 2024 (invited, accepted).
- Clements N, Esplen N, Bateman J, Dosanjh M, Korysko P, Farabolini W, Corsini R, Bazalova-Carter M, Mini-GRID radiotherapy on the CLEAR very-high-energy electron beamline: collimator optimization, film dosimetry, and Monte Carlo simulations, Phys Med Biol 2024 (055003).
- Hart A, Giguère C, Bateman J, Korysko P, Farabolini W, Rieker V, Esplen N, Corsini R, Dosanjh M, Beaulieu L, Bazalova-Carter M, Plastic scintillator dosimetry of ultrahigh dose-rate 200 MeV electrons at CLEAR, IEEE Sensors 2024 (14229-14237).
- Esplen N, Egoriti L, Planche T, Rädel S, Koay H-W, Humphries B, Ren X, Ford N, Hoehr C, Gottberg A, Baza
lova-Carter M , Dosimetric characterization of a novel UHDR megavoltage x-ray source for FLASH radiobiological experiments, Sci Rep 2024 (14:822). Highlighted by Physics World. - Clements N, Esplen N, Bazalova-Carter M, A feasibility study of ultra-high dose rate mini-GRID therapy using very high-energy electron beams for a simulated pediatric brain case, Phys Med 2023 (102637).
- Ren X, Egoriti L, Esplen N, Rädel S, Humphries B, Koay H-W, Planche T, Hoehr C, Gottberg A, Bazalova-Carter M, Ford N, Using in vivo respiratory-gated micro-computed tomography imaging to monitor pulmonary side effects in 10 MV FLASH and conventional radiotherapy, Proc. SPIE 12468, Medical Imaging 2023: Biomedical Applications in Molecular, Structural, and Functional Imaging 2023 (conference proceedings).
- Clements N, Bazalova-Carter M, Esplen N, Monte Carlo optimization of a GRID collimator for preclinical megavoltage ultra high dose-rate spatially-fractionated radiation therapy, Phys Med Biol 2022 (185001).
- Shaharuddin S, Hart A, Bazalova-Carter M, Beaulieu L, Giguere C, Kleefeld C, and Foley MJ, Evaluation of scintillation detectors for ultrahigh dose-rate x-ray beam dosimetry, Proc. SPIE 2022; 170-177 (conference proceedings).
- Hart A, Cecchi D, Giguère C, Larose F, Therriault-Proulx F, Esplen N, Beaulieu L, Bazalova-Carter M, Lead-doped scintillator dosimeters for detection of ultrahigh dose-rate x-rays, Phys Med Biol 2022 (105007).
- Esplen N, Egoriti L, Palley B, Planche T, Hoehr C, Gottberg A, and Bazalova-Carter M, Design optimization of an electron-to-photon conversion target for ultra-high dose rate x-ray (FLASH) experiments at TRIUMF, Phys Med Biol 2022 (105003).
- Shaharuddin S, Hart A, Cecchi D, Bazalova-Carter M and Foley M, Real-time dosimetry of ultrahigh dose-rate x-ray beams using scintillation detectors, IEEE Sensors 2021; 1-4 (conference proceedings).
- Cecchi D, Therriault-Proulx F, Lambert-Girard S, Hart A, MacDonald A, Pfleger M, Lenckowski M, Bazalova-Carter M, Characterization of an x-ray tube-based ultrahigh dose-rate system for in vitro irradiations, Med Phys 2021; 7399-7409.
- Bazalova-Carter M and Esplen N, On the capabilities of conventional x-ray tubes to deliver ultra-high (FLASH) dose rates, Med Phys 2019; 5690-5695 (Medical Physics Letter, Editor's Choice, Highlighted on physicsworld.com).
Kilovoltage Optimized AcceLerator for Adaptive (KOALA) therapy
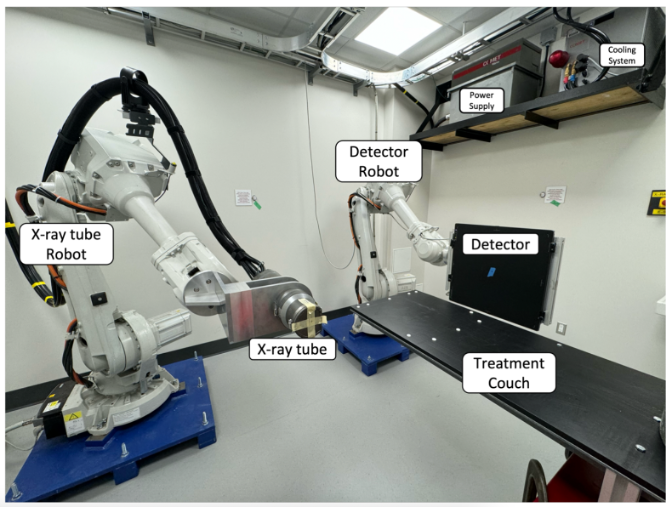
Access to radiotherapy therapy is limited in low- and middle-income countries (LMIC). In some LMIC <10% of eligible patients have access to radiotherapy due to its high cost.The standard of RT care is the delivery of high‐energy (6–15 MV) photons, generated by a medical linear accelerator (linac), to the site of disease. Linacs utilize a number of expensive technologies, such as precisely machined waveguides and high‐voltage generators, to accelerate electrons to relativistic speeds. Upon collision with a tungsten target these electrons produce megavoltage (MV) bremsstrahlung photons, which are filtered, collimated, and directed to the site of treatment. The high energy of these photons necessitates expensive treatment infrastructure known as "vaults" to house the linac in order to shield medical personnel from radiation. The result of this high cost is strain on healthcare systems.
The goal of this project is to design a low-cost radiotherapy device that uses kilovoltage x-rays. Compared the linac-driven megavoltage x-rays, high beam attenuation and low beam output have to be overcome. So far, we have demonstrated proof of principle by means of Monte Carlo simulations and some limited experiments on phantoms. In collaboration with McGill University, we have developed KVAT treatment planning optimization. We have demonstrated that the most suitable candidates for KVAT treatments might be breast and lung patients, but not prostate patients. Our industrial partner Precision RT (Las Vegas, NV) is currently testing the first machine prototype and we are assisting with the experimental validation.
Related publications- O'Connell J, Weil MD, Bazalova-Carter M, Non-coplanar lung SABR treatments delivered with a gantry-mounted x-ray tube, Phys Med Biol 2024 (025002).
- Stalbaum T, Boyd D, Weil M, Chen H, Plies M, Song S, Ziskin V, Bazalova-Carter M, Boone J, Daly M, Partain L, 200 kV x-ray source for radiotherapy and imaging: preliminary results and discussion, Proc. SPIE 11312, Medical Imaging 2020: Physics of Medical Imaging 2020 (conference proceedings).
- Breitkreutz DY, Renaud MA, Weil MD, Zavogordni S, Han E, Baxter H, Seuntjens J, Song S, Boyd D and Bazalova-Carter M, Monte Carlo calculated kilovoltage x-ray arc therapy plans for three lung cancer patients, BPEX 2019; 065022.
- Breitkreutz DY, Renaud M-A, Seuntjens JP, Weil MD, Zavgorodni SF, Bazalova-Carter M, Inverse optimization of low-cost kilovoltage x-ray arc therapy plans, Med Phys 2018; 5161-5171.
- Breitkreutz D , Weil MD, Zavgorodni S, Bazalova-Carter M, Monte Carlo simulations of a kilovoltage external beam radiotherapy system on phantoms and breast patients. Med Phys 2017: 6548-6559.
- Bazalova-Carter M, Weil MD, Breitkreutz D, Wilfley BP, and Graves E, Feasibility of external beam radiation therapy to deep-seated targets with kilovoltage x-rays, Med Phys 2017; 45: 597-607.
X-ray imaging with photon-counting detectors
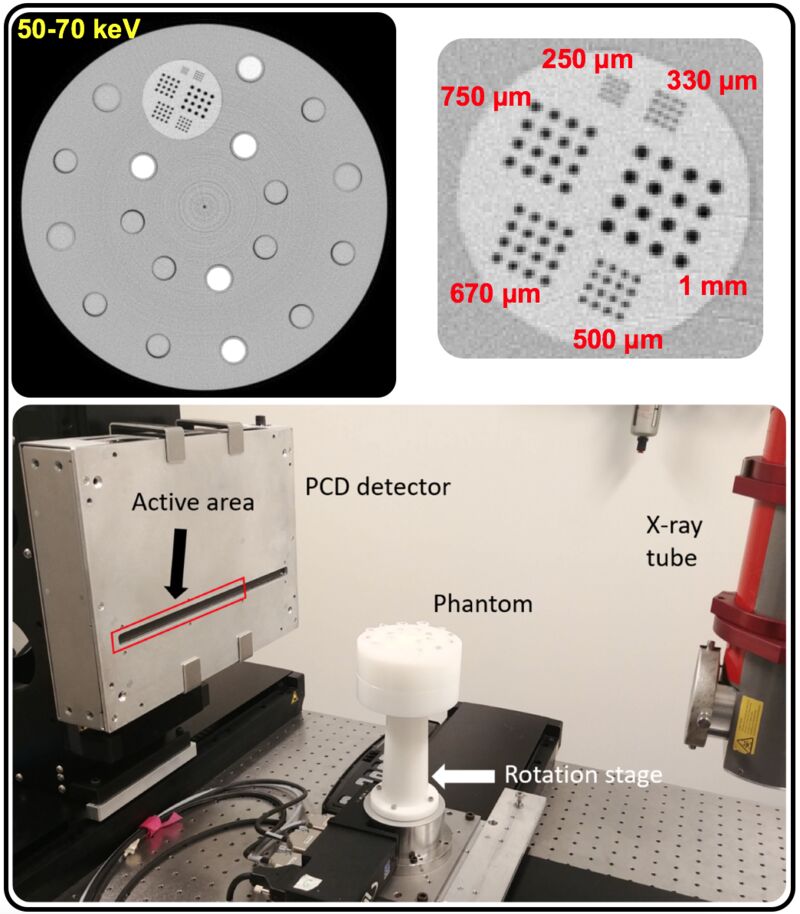
We investigate novel x-ray CT imaging modalities for improved cancer diagnosis and treatment by integrating photon-counting detectors into our table-top x-ray imaging system. We have two streams of research in this area: 1) x-ray fluorescence CT (XFCT) and 2) photon-counting CT imaging (PCCT). Both modalities can be used for the visualization of high-atomic number materials attached to agents targeting a specific site or molecular processes.
XFCT is a promising imaging modality for visualization of high atomic number contrast agents by detecting the x-ray scattered beam using photon-counting detectors with high energy resolution (1-2%). We have performed Monte Carlo simulations of XFCT imaging using a number of different detectors, as well as simulations of XFCT imaging with various excitation beams. We have also experimentally verified our results with Amptek CdTe detectors using a number of contrast agents (I, Gd, Au).
Since October 2018, we have collaborated with Redlen Technologies on photon-counting CT imaging. We currently have two CZT detectors of various areas with 330µm pixel pitch that we are testing for PCCT of high-atomic contrast agents, such as gold or lanthanide nanoparticles. Thanks to our collaboration with Prof. van Veggel's group in the UVic Chemistry department, we have been able to evaluate the capability of our CZT detector to image lanthanide contrast agents very close in atomic number.
We have been using our PCCT detectors extensively for enhanced contrast x-ray CT imaging, material extraction as well as for their superior spatial resolution. In a number of publications we have demonstrated that high-resolution images of coronary stents can be acquired with our system and accurate material segmentaiton in terms of atomic number and mass density can be achieved.
Related publications
- Rodesch P-A, Richtsmeier D, Murphy K, K Iniewski and Bazalova-Carter M, Photon-counting detector step-wedge calibration enabling water and iodine material decomposition, JINST 2024 (P05030).
- Richtsmeier D, Rodesch P-A, K Iniewski and Bazalova-Carter M, Material decomposition with a prototype photon-counting detector CT system: expanding a stoichiometric dual-energy CT method via energy bin optimization and K-edge imaging, Phys Med Biol 2024 (055001).
- Richtsmeier D, Rodesch P-A, Siu W, Iniewski K, Bazalova-Carter M, The feasibility of accurate stent visualization with photon-counting detector CT and K-edge imaging, IEEE TRPMS 2023; 712 - 718.
- Laidlaw J, Earl N, Shavdia N, Davis R, Mayer S, Karaman D, Rodesch P-A, Richtsmeier D, Bazalova-Carter M, Design and CT imaging of Casper, an anthropomorphic breathing thorax phantom, BPEX 2023 (025008).
- Rodesch P-A, Richtsmeier D, Iniewski K, Bazalova-Carter M, Comparison of threshold energy calibrations of a photon-counting detector and impact on CT reconstruction, IEEE TRPMS 2023; 263 - 272.
- O'Connell J, Kundu S, Saidaminov M, Bazalova-Carter M, Next Generation High Resolution Perovskite Direct Conversion Detector: Monte Carlo Design Optimisation and Virtual Clinical Trial, Phys Med Biol 2023 (025016). Highlighted by Physics World.
- Richtsmeier D, O'Connell J. Rodesch P-A, K Iniewski and Bazalova-Carter M, Metal artifact correction in photon-counting detector CT: metal trace replacement using high-energy data, Med Phys 2023; 380-396.
- Nguyen J, Richtsmeier D, Iniewski K and Bazalova-Carter M, Contaminant Detection using a CZT photon counting detector with TDI image reconstruction, JINST 2022; P05010.
- Clements N, Richtsmeier D, Hart A, Bazalova-Carter M, Multi-contrast CT imaging using a high energy resolution CdTe detector and a CZT photon-counting detector, JINST 2022; P01004.
- Nguyen J, Rodesch P-A, Richtsmeier D, K Iniewski and Bazalova-Carter M, Optimization of a CZT photon counting detector for contaminant detection, JINST 2021; P11015.
- Tanguay J, Richtsmeier D, Dydula C, Day JA, Iniewski K, Bazalova-Carter M, A Detective Quantum Efficiency for Spectroscopic X-ray Imaging Detectors, Med Phys 2021; 6781-6799.
- Richtsmeier D, Guliyev E, Iniewski K, Bazalova-Carter M, Contaminant detection in non-destructive testing using a CZT photoncounting detector JINST 2021; P01011.
- Richtsmeier D, Dunning CAS, Kris Iniewski, Bazalova-Carter M, Multi-contrast K-edge imaging on a bench-top photon-counting CT system: Acquisition parameter study, JINST 2020; P10029.
- Dunning C and Bazalova-Carter M, Design of a Combined X-ray Fluorescence Computed Tomography (CT) and Photon-Counting CT Table-top Imaging System, JINST 2020; P06031.
- Dunning C, O'Connell J, Robinson S, Murphy K, Frencken A, Van Veggel F, Iniewski K, and Bazalova-Carter M Photon-counting computed tomography of lanthanide contrast agents with a high-flux 330-μm pitch cadmium zinc telluride (CZT) detector in a table-top system, SPIE JMI 2020; 033502.
- O'Connell J, Murphy K, Robinson S, Iniewski K, and Bazalova-Carter M, Unsupervised Learning Methods in X-ray Spectral Imaging Material Segmentation, JINST 2019; P06022.
- Dunning C and Bazalova-Carter M, X-ray Fluorescence Computed Tomography Induced by Photon, Electron, and Proton Beams, IEEE Trans Med Im 2019; 2735-2743.
- O'Connell J, Iniewski K, and Bazalova-Carter M, Optimal Planar X-ray Imaging Soft Tissue Segmentation Using a Photon Counting Detector, JINST 2019; P01020.
- Dunning C and Bazalova-Carter M, Optimization of a table-top x-ray fluorescence computed tomography (XFCT) system, Phys Med Biol 2018; 235013.
- Dunning C and Bazalova-Carter M, Sheet beam x-ray fluorescence computed tomography (XFCT) imaging of gold nanoparticles, Med Phys 2018; 2572-2582.
Small animal radiotherapy
We are interested in improvements of dose delivery accuracy performed with image-guided small animal irradiators, such as with the small animal radiation research platform (SARRP) by Xstrahl, Inc and the small animal radiotherapy (SmART) system by PXi. To this end, we have performed a multi-institutional study on the image quality and guidance of the two systems. In collaboration with Oxford and Xstrahl, we have developed and evaluated a 3D-printed phantom for small animal image quality and targeting assessment.

We have also designed a 3D-printed phantom for evaluation of dosimetry. This phantom was built based on a microCT scan of a mouse with a subcutaneous tumor on its right hind leg. The phantom was 3D printed with two different materials mimicking soft tissue and bone. The bone material was only slightly different than the soft tissue material and provided mainly visual contrast rather than CT contrast. The lungs were excavated and filled with polystyrene to mimic lung tissue.
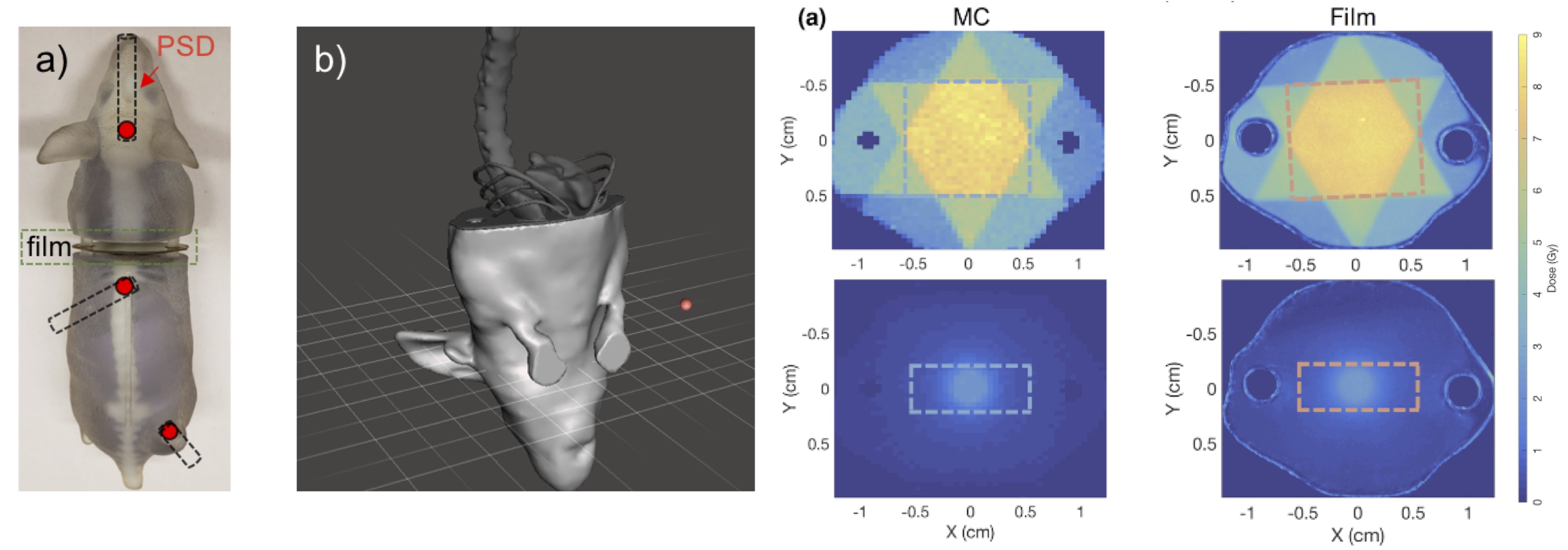
The phantom was equipped with two different dosimeters to enable dose measurements that was tested with a number of treatment scenarios on the SARRP.The phantom was split into two pieces in order to allow for an insertion of a laser-cut Gafchromic film for measurements of 2D doses. Dose distributions measured by the film agreed well with Monte Carlo calculated doses. Additionally, three holes in the brain, abdomen and in the orthotopic tumors were included in the model for the insertion of a plastic scintillator dosimeter (PSD). Thanks to our collaboration with François Therriault-Proulx of MedScint and Luc Beaulieu of Laval University we were able to evaluate the use of their PSD to small animal radiotherapy. We then used it for dose evaluation in these three sites for various treatment setups and found a good agreement with Monte Carlo simulations.
Related publications- Johnstone C and Bazalova-Carter M, A review of small animal dosimetry techniques: image-guided and spatially-fractionated therapy, Journal of Physics: Conference Series 2023 (012013).
- Ren X, Egoriti L, Esplen N, Rädel S, Humphries B, Koay H-W, Planche T, Hoehr C, Gottberg A, Bazalova-Carter M, Ford N, Using in vivo respiratory-gated micro-computed tomography imaging to monitor pulmonary side effects in 10 MV FLASH and conventional radiotherapy, Proc. SPIE 12468, Medical Imaging 2023: Biomedical Applications in Molecular, Structural, and Functional Imaging 2023 (conference proceedings).
- Mahuvava C, Esplen N, Poirier Y, Kry SF, Bazalova-Carter M, Dose calculations for pre-clinical radiobiology experiments conducted with single-field cabinet irradiators, Med Phys 2022; 1911-1923.
- Poirier Y, Anvari A, Johnstone CD, Brodin NP, Dos Santos M, Bazalova-Carter M, Sawant A, A Failure Modes and Effects Analysis Quality Management Framework for Image-Guided Small Animal Irradiators : A Change in Paradigm for Radiation Biology, Med Phys 2020; 2013-2022.
- Esplen N, Therriault-Proulx F, Beaulieu L and Bazalova-Carter M, Preclinical dose verification using a 3D printed mouse phantom for radiobiology experiments, Med Phys 2019; 5294-5303.
- Breitkreutz DY, Bialek S, Vojnovic B, Kavanagh A, Johnstone CD, Rovner Z, Tsouchlos P, Kanesalingam T, Bazalova-Carter M , A 3D-printed modular phantom for quality assurance of image-guided small animal irradiators: design, imaging experiments and Monte Carlo simulations, Med Phys 2019; 2015-2024.
- Esplen N, Alyaqoub E and Bazalova-Carter M, Technical Note: Manufacturing of a realistic mouse phantom for dosimetry of radiobiology experiments, Med Phys 2019; 1030-1036.
- Johnstone C, Therriault-Proulx F, Beaulieu L, and Bazalova-Carter M, Characterization of a Plastic Scintillating Detector for the Small Animal Radiation Research Platform (SARRP), Med Phys 2019; 394-404.
- Johnstone C and Bazalova-Carter M, MicroCT Imaging Dose to Mouse Organs using a Validated Monte Carlo Model of the Small Animal Radiation Research Platform (SARRP), Phys Med Biol 2018; 115012.
- Johnstone C, Lindsay P, Graves EE, Wong E, Perez J, Poirier Y, Ben-Bouchta Y, Kanesalingam T, Chen H, Rubinstein A, Sheng K, Bazalova-Carter M, Multi-Institutional MicroCT Image Comparison of Image-Guided Small Animal Irradiators, Phys Med Biol 2017: 5760-5776.